Overview
Broadly, my research integrates physiological and mechanical principles to understand how animal form relates to function.
Physiology and mechanics apply real constraints to organisms, which they manage and exploit through adaptation (evolution) and plasticity (development and learning).
Currently, I am studying how patterns in locomotion emerge from optimization processes. A prevailing hypothesis posits that organisms often choose locomotion patterns minimizing effort (metabolic energy expenditure), while fulfilling some relevant task- e.g. foraging, migrating or tracking. Through modelling and experiment, I have been putting this hypothesis to the test.
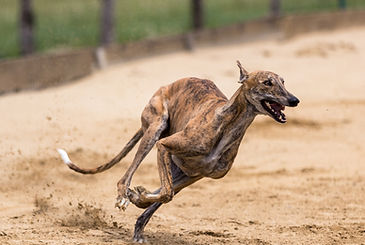
Current Research
Muscles, Reaching, and Ageing
In my current post, I'm studying how muscle contractile properties change with age in a mouse model.
I'm also exploring a simple model of a human arm to connect these muscle property changes to variation in performance.
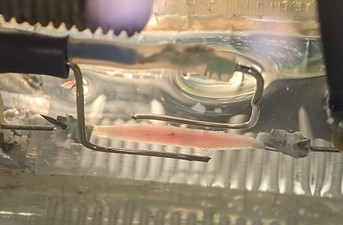
Optimal Gearing Hypothesis
In collaboration with David Labonte (Imperial College London), I'm exploring how gearing can affect the kinetic energy output of musculoskeletal systems- and whether animals adopt these optima.
We find that the optimum changes depending on the muscle properties and shape, the load relative to strength, and the mechanical environment- leading to a rich scope for diversification! ICB paper

Past Research
Gait transitions in trackways
On the DAWNDINOS project, I collected data on fossil trackways and generated a model of Rauisuchian locomotion. We proposed that the aggregate trackway data represent a gait transition in Chirotheriid trackmakers. Read the paper here

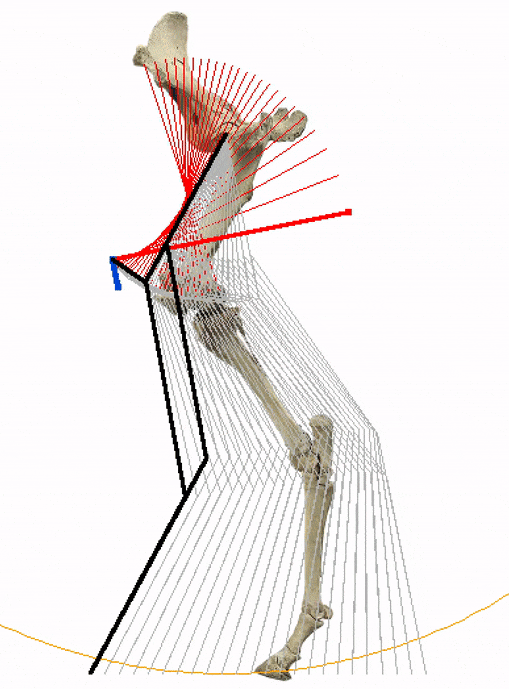
Work avoidance through serial linkages
Recent work by Jim Usherwood demonstrates how muscles can act like spokes on a bicycle wheel. Muscles take turns tensioning the leg and forming serial linkages- in a way that reduces the required work
I demonstrated how this effect could work in the sprawled, plantigrade locomotion of lizards SEB Talk
Minimally-constrained model of legged locomotion
Conceptual models in locomotion vary from extremely abstract (e.g. a single point mass on a stick) to extremely complex (e.g. high-fidelity OpenSim musculoskeletal models). I developed a model in the balance; sufficiently complex to make precise predictions about behaviour (e.g. walk-run transition speeds, preferred gaits) but simple enough to understand underlying mechanisms, and applicable to a broad range of organisms.
I describe the model in PLOS Computational Biology. Consisting of four "pistons" connected to a rigid body, it accurately predicts walking at slow speeds and trotting at intermediate speeds and the walk-trot transition speed for dogs. Importantly, this model has no springs, suggesting that elastic tendons are not a prerequisite for the economy of these strategies.
A follow up paper showed how different ways of accounting for effort in locomotion lead to different (and sometimes, bizarre) optimal gaits Frontiers paper
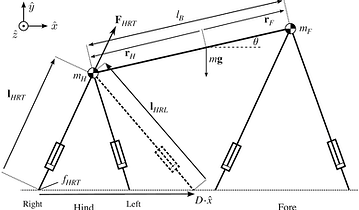
The Pitch-Translation Tradeoff Hypothesis
Why do mammals trot? This "two-beat" gait involves expensive up-down oscillation of the body, that could be mitigated with distributed "four-beat" gaits. Yet trotting is the slow-running gait of choice for most mammals - though some, like giraffes, wildebeest and elephants, prefer not to trot.
I have been exploring whether the pitch moment of inertia resolves this conundrum. Pitching the body is expensive, and can be avoided with a trot. But if your body naturally resists pitching (e.g. a giraffe's long neck), then you can use alternatives to trotting.
I have validated this theory in a modelling framework (JEB paper, talk) and am looking to perform experiments and measurements to verify it in animals.
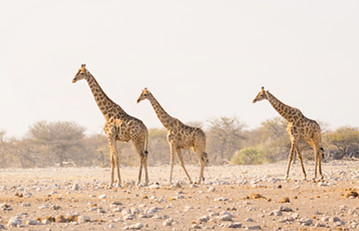
Reduced gravity locomotion
To determine if animals choose gaits to optimize metabolic energy expenditure, we can modify their environment to make the usual pattern suboptimal, while an unusual pattern becomes more economical.
Changing gravity is a pronounced environmental change with a reliable effect on an organism's mechanics. With simple conceptual models, we can make specific predictions about how humans and other animals will adapt for economical locomotion.
JEB paper, Dynamic Walking Poster, Summary Video, 2019 Longer Video, 2020 Update Video
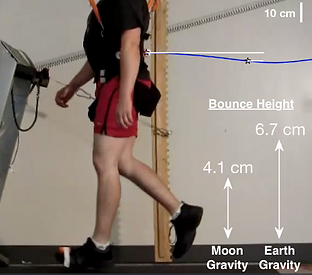
Insect wing microstructure for efficient drop shedding
Insect wings are covered in small bumps, hairs or scales called microstructures. These microstructures often have a particular but locally-variable orientation. Why? For my undergraduate independent research project, I showed that the orientation may promote efficient drop shedding. Published in PLOS One

Wing rapid area change for fast perching
Many birds go from flying at a high speed to landing on a small branch in fractions of a second. One way they may accomplish this is by quickly changing the frontal area of their wings. This "rapid area change" takes advantage of strange added mass effects, quickly shedding the mass of the air attached to the wing like propellant firing out of a rocket nozzle.
Published: J Fluid Mech, Bioinsp Biomim. See also my tutorial on added mass recovery
